Difference between revisions of "LaserCooling"
(→Overview) |
(→References) |
||
(160 intermediate revisions by 6 users not shown) | |||
Line 1: | Line 1: | ||
− | + | In the laser cooling lab, we seek to study ultracold molecules by first loading molecules into a magneto-optical trap (MOT) and then transferring these molecules to a magnetic trap for further cooling. Interesting later experiments could involve studying atom-molecule or molecule-molecule collisions, as well as using the ultracold sample of diatomic molecules for quantum simulation or precision measurement experiments. | |
− | + | [[File:motcoils.jpg|thumb|300px|AC MOT coils]] | |
− | + | ||
− | + | ||
− | === | + | == Research Overview == |
− | + | ||
− | + | ||
− | + | ||
− | === | + | <html> |
− | + | <iframe style="padding:6px;" width="400" height="244" src="//www.youtube.com/embed/xAsiwVdpU1w" frameborder="0" allowfullscreen align = "right"></iframe></td> </html> The goal of this experiment is to load a magneto-optical trap (MOT) with the diatomic radical calcium monofluoride (CaF) using a two-stage buffer-gas beam source (for details on buffer-gas cells see [[#References|[1-3]]]). We first ablate a solid precursor of atomic Ca with a pulsed Nd:YAG laser. We simultaneously flow sulfur hexafluoride (SF<sub>6</sub>) into the buffer-gas cell, leading to a chemical reaction which produces CaF. The hot molecular gas then thermalizes with ~1 K Helium buffer-gas and is extracted into a beam. The molecular beam has an average forward velocity of 50-60 m/s out of our two-stage cell. While such velocities are low enough to load conventional atomic MOTs ([[#Buffer-Gas Loaded MOTs for Ytterbium, Thulium, Erbium and Holmium|see our previous work on lanthanide atoms]]), the estimated capture velocity for a MOT of CaF is less than 10 m/s. A slowing stage is thus required to bring a sufficient number of molecules to below the capture velocity. We use a white-light slowing technique for this beam deceleration, as was demonstrated in our recent paper [[#References|[14]]]. An additional challenge to trapping molecules is the existence of magnetic dark states in molecules, which arise due to the fact that we trap the molecules on a transition with "inverted" angular momentum structure. We address this problem by switching the polarization and the magnetic field of the MOT very rapidly (~1 MHz) to depopulate those dark states ([[#Laser Cooling and Trapping of Diatomic Radicals (CaF)|see below for details]]). | |
− | |||
− | == | + | ==Latest News== |
− | + | === (August 2016) CaF slowing results published in J. Phys. B === | |
− | + | Recently, we have accomplished laser slowing of CaF molecules from a two-stage buffer gas beam source down to longitudinal velocities of approximately 5 m/s. Our scheme uses three lasers (one main transition and two for repump transitions) which are spectrally broadened to address the velocity spread of the source as well as the hyperfine structure of the molecules. Our work on laser slowing of CaF has been published in ''J. Phys. B: At. Mol. Opt. Phys.'' for a special issue on "Atomic and molecular processes in the ultracold regime, the chemical regime, and astrophysics." [http://iopscience.iop.org/article/10.1088/0953-4075/49/17/174001/meta You can find the paper here.] | |
+ | [[File:Slowing_data_CaF.jpg|thumb|center|500px|Demonstration of slowing of our molecular beam via radiation pressure force.]] | ||
− | + | === (November 2015) Eunmi defends her thesis === | |
+ | Congratulations to Eunmi Chae for successfully defending her thesis! Eunmi will continue her work on laser slowing and cooling of CaF for a few months before starting a post doc in the Takahashi group at Kyoto. | ||
+ | |||
+ | === (Summer 2014) AC-MOT of Lithium === | ||
[[File:Li_ac_dc_mot_comparison.png|thumb|left|300px|Comparison of a DC- and AC-MOT for Li on the D1 line.]] | [[File:Li_ac_dc_mot_comparison.png|thumb|left|300px|Comparison of a DC- and AC-MOT for Li on the D1 line.]] | ||
+ | [[File:20140312_Li_DC_MOT.jpg|thumb|300px|Lithium MOT using our in-vacuum coils.]] | ||
+ | |||
+ | |||
+ | |||
+ | We realized an AC-MOT for Lithium-6 on the D1 line. See the picture on the right for an image of the MOT in action. This transition has magnetic dark states which reduce the efficiency of a regular DC-MOT configuration. A switching of the polarization and the magnetic field actively remixes the Zeeman substates and keeps the atom in the cooling cycle. Three different time traces comparing the beam signal without trapping fields, a DC-MOT and an AC-MOT are shown in the left figure. Both the magnetic field coils and the polarization of the molasses laser beams are switched at a frequency of 6 MHz. The improvement in the lifetime using the AC configuration is quite evident. For further details on AC-MOTs see e.g. [http://dx.doi.org/10.1103/PhysRevLett.110.143001 Phys. Rev. Lett. 110, 143001 (2013)] and [http://dx.doi.org/10.1103/PhysRevLett.101.173201 Phys. Rev. Lett. 101, 173201 (2008)]. | ||
+ | |||
+ | === (June 2014) Atomic MOTs paper accepted in New Journal of Physics === | ||
+ | |||
+ | Our paper [http://iopscience.iop.org/1367-2630/16/6/063070 Buffer-gas loaded magneto-optical traps for Yb, Tm, Er and Ho] has been accepted in New Journal of Physics. | ||
+ | |||
+ | <!--=== Loaded magneto-optical trap for Yb with our in-vacuum coils ===--> | ||
+ | |||
+ | <!--[[File:YbMOTSlowing.jpg|thumb|200px|Yb MOT using in-vacuum coils.]]--> | ||
+ | |||
+ | <!--As a first test with our new apparatus and our in-vacuum coils, we loaded an Ytterbium MOT (picture on the right).--> | ||
+ | |||
+ | |||
+ | ==People== | ||
+ | |||
+ | |||
+ | |||
+ | |||
+ | '''Grad Students''' | ||
+ | * Loic Anderegg | ||
+ | * Ben Augenbraun | ||
+ | |||
+ | '''Former Students and Postdocs''' | ||
+ | * Aakash Ravi | ||
+ | * Eunmi Chae | ||
+ | * Boerge Hemmerling | ||
+ | * Nick Hutzler | ||
+ | * Garrett Drayna | ||
+ | |||
+ | ===Collaborators=== | ||
+ | * Jun Ye (PI), Matthew Hummon, Mark Yeo, Alejandra Collopy, Yong Xia | ||
+ | |||
+ | [http://jila.colorado.edu/yelabs/research/ultracold-molecules Ye Group Ultracold Molecules Website] | ||
+ | |||
+ | * Wolfgang Ketterle (PI) | ||
+ | |||
+ | [http://cua.mit.edu/ketterle_group/home.htm Ketterle Group Website] | ||
− | |||
− | |||
− | == | + | == Detailed Overview of Experiment == |
+ | [[File:CaF_slowing_layout.jpg|thumb|300px|Setup for slowing CaF molecules from a cryogenic buffer gas beam source.]] | ||
− | + | [[File:CaF_Level_scheme.jpg|thumb|300px|CaF laser cooling scheme. Transitions for a two-photon fluorescence detection of molecules are shown on the left within the grey box. (Inset) X state hyperfine structure.]] | |
− | + | We are investigating the laser slowing and magneto-optical trapping of diatomic molecules. Laser cooling of molecules requires buffer-gas cooling to produce samples of ground-state molecules which have necessary rotational phase space densities for current molecular laser cooling schemes. We use slow beam technology developed in our group [[#References|[1-3]]] to reduce the amount of kinetic energy and number of cycled photons needed to bring molecules to rest in a 3D trap. | |
− | [[ | + | Currently, our group is working with the radical CaF. We have produced a slow, cryogenic beam of CaF with rotational cooling and we see slowing of molecules down to longitudinal velocities of ~ 5 m/s, following a scheme similar to the one used in [[#References|[3-6]]]. |
− | + | Other groups working on laser cooling and slowing of diatomic molecules include: | |
− | + | * CU Boulder: Yttrium oxide (YO). [http://jila.colorado.edu/yelabs/research/ultracold-molecules Jun Ye Group Ultracold Molecules Website] | |
− | + | * Yale University: Strontium fluoride (SrF). [http://www.yale.edu/demillegroup/index.html Dave DeMille Group Website] | |
− | [ | + | * Calcium Fluoride: Calcium fluoride (CaF). [http://www3.imperial.ac.uk/ccm/research/coldmol/laser_cooling Ed Hinds Group Website] |
− | + | === Buffer-Gas Loaded MOTs for Ytterbium, Thulium, Erbium and Holmium === | |
− | [http://arxiv.org/ | + | <!--[[File:Yb174 399nm MOT.jpg|left|thumb|200px|Yb MOT on the 400 nm transition in our experiment.]]--> |
+ | [[File:BGMOTapparatus.png|right|thumb|800px|Schematic of the buffer-gas loaded MOT experiment (from [http://arxiv.org/abs/1310.3239 Buffer-gas loaded magneto-optical traps for Yb, Tm, Er and Ho]).]] | ||
+ | As a proof of principle for loading MOTs from a buffer-gas beam source, we first investigated laser cooling and trapping of atoms. We made the first buffer-gas loaded MOT using Yb atoms. A schematic of the apparatus can be seen on the right. This MOT, which used no slowing of any kind (Zeeman slower, slowing laser, etc.), demonstrated the feature of our buffer gas beam technology, which we plan to use for a molecular MOT. Furthermore, this MOT demonstrated that, in principle, buffer-gas beam technology is compatible with 3D magneto-optical trapping. Owing to the universal nature of this source, we implemented MOTs for the lanthanides ytterbium, thulium, erbium and holmium-- each requiring only a change of the ablation pre-cursor target and the MOT laser frequency. These highly magnetic species are of interest for quantum simulation and quantum computation. They are also refractory elements and have low loading rates due to the difficulties with oven sources. Our buffer-gas loaded MOTs circumvent these high temperature oven sources and allow loading rates over 10^8 /sec/shot, or over 10^9/sec cumulative. This technology could be used to co-load multiple species with little technological overhead (e.g., no multiple Zeeman slowers). We also demonstrate this flexibility in the case of ytterbium by loading many different isotopes into the MOT by adjusting the detuning of the MOT laser frequency only. Below is a video which shows a picture of the Yb MOT fluorescence with the detuning being changed between the frames. | ||
− | + | <!--[[File:Yb_isotope_mots.ogg|thumb|200px|Loading of Yb isotopes by tuning the MOT wavelength]]--> | |
− | + | Our work on these lanthanide atoms is summarized in the following article: | |
− | + | [http://iopscience.iop.org/1367-2630/16/6/063070 Buffer-gas loaded magneto-optical traps for Yb, Tm, Er and Ho]. B. Hemmerling, G. K. Drayna, E. Chae, A. Ravi, and J. M. Doyle, New Journal of Physics 16, 063070 (2014). | |
+ | <!--[[File:BGMOTapparatus2.png|right|thumb|482px|Schematic of the second generation buffer-gas loaded MOT experiment.]]--> | ||
+ | ==== Video of Ytterbium isotope MOTs ==== | ||
+ | The solid precursor used for ablation contains the natural abundance of Yb isotopes, i.e. 3% <sup>170</sup>Yb, 14% <sup>171</sup>Yb, 22% <sup>172</sup>Yb, 16% <sup>173</sup>Yb, 32% <sup>174</sup>Yb and 13% <sup>176</sup>Yb. The ablation process, as opposed to photoionization, lacking an isotope-selective character brings each isotope into the gas phase where it is subsequently cooled by the He buffer gas. Due to the absence of a Zeeman slower, each element can be loaded into the MOT by only correcting for the isotope shift in the frequency detuning of the molasses laser. The top graph in the video shows the in-cell absorption spectrum and the change in laser frequency (The frequencies given in the video are measured with our wavemeter and thus are NOT precision measurements of the frequencies for the Yb transitions. For precise values, the reader is e.g. referred to [http://dx.doi.org/10.1103/PhysRevA.82.043408 PRA 82, 043408 (2010)].) Note, that one of the isotopes, <sup>173</sup>Yb, does not form a magneto-optical trap. This is due to the presence of magnetic dark states. | ||
+ | <html><iframe width="480" height="293" src="//www.youtube.com/embed/2b7lfarW9i8" frameborder="0" allowfullscreen></iframe></html> | ||
== References == | == References == | ||
− | + | #N. R. Hutzler, H.-I Lu, and J. M. Doyle, '''The Buffer Gas Beam: An Intense, Cold, and Slow Source for Atoms and Molecules''', [http://dx.doi.org/10.1021/cr200362u Chem. Rev., 112, 4803 (2012)] | |
− | + | #H.-I Lu, J. Rasmussen, M. J. Wright, D. Patterson, and J. M. Doyle, '''A cold and slow molecular beam''', [http://dx.doi.org/10.1039/c1cp21206k Phys. Chem. Chem. Phys. 13, 18986 (2011)] | |
− | + | #D. Patterson and J. M. Doyle, '''Bright, Guided, molecular beam with hydrodynamic enhancement''', [http://dx.doi.org/10.1063/1.2717178 J. Chem. Phys. 126, 154307 (2007)] | |
− | + | #E. S. Shuman, J. F. Barry, D. R. Glenn, and D. DeMille, '''Radiative Force from Optical Cycling on a Diatomic Molecule''', [http://dx.doi.org/10.1103/PhysRevLett.103.223001 Phys. Rev. Lett. 103, 223001 (2009)] | |
− | + | #E. S. Shuman, J. F. Barry, and D. DeMille, '''Laser cooling of a diatomic molecule''', [http://dx.doi.org/10.1038/nature09443 Nature 467, 820 (2010)] | |
+ | #M. T. Hummon, M. Yeo, B. K. Stuhl, A. L. Collopy, Y. Xia, and J. Ye, '''2D Magneto-Optical Trapping of Diatomic Molecules''', [http://dx.doi.org/10.1103/PhysRevLett.110.143001 Phys. Rev. Lett. 110, 143001 (2013)] | ||
+ | #M. Harvey, A. J. Murray, '''Cold Atom Trap with Zero Residual Magnetic Field: The ac Magneto-Optical Trap ''', [http://dx.doi.org/10.1103/PhysRevLett.101.173201 Phys. Rev. Lett. 101, 173201 (2008)] | ||
+ | #V. Zhelyazkova, A. Cournol, T. E. Wall, A. Matsushima, J. J. Hudson, E. A. Hinds, M. R. Tarbutt, and B. E. Sauer, '''Laser cooling and slowing of CaF molecules ''', [http://dx.doi.org/10.1103/PhysRevA.89.053416 Phys. Rev. A. 89, 053416 (2014)] | ||
+ | #B. Hemmerling, G. K. Drayna, E. Chae, A. Ravi, J. M. Doyle, '''Buffer gas loaded magneto-optical traps for Yb, Tm, Er and Ho''', [http://iopscience.iop.org/1367-2630/16/6/063070 New J. Phys. 16, 063070 (2014)] | ||
+ | #J. F. Barry, D. J. McCarron, E. B. Norrgard, M. H. Steinecker, and D. DeMille, '''Magneto-optical trapping of a diatomic molecule''', [http://dx.doi.org/10.1038/nature13634 Nature 512, 286 (2014)] | ||
+ | #D. J. McCarron, E. B. Norrgard, M. H. Steinecker, D. DeMille, '''Improved magneto-optical trapping of a diatomic molecule''', [http://dx.doi.org/10.1088/1367-2630/17/3/035014 New J. Phys. 17, 035014 (2015)] | ||
+ | #M. Yeo, M. T. Hummon, A. L. Collopy, B. Yan, B. Hemmerling, E. Chae, J. M. Doyle, J. Ye, '''Rotational state microwave mixing for laser cooling of complex diatomic molecules''', [http://journals.aps.org/prl/abstract/10.1103/PhysRevLett.114.223003 Phys. Rev. Lett. 114, 223003 (2015)] | ||
+ | #E. B. Norrgard, D. J. McCarron, M. H. Steinecker, M. R. Tarbutt, and D. DeMille, '''Submillikelvin Dipolar Molecules in a Radio-Frequency Magneto-Optical Trap''', [http://dx.doi.org/10.1103/PhysRevLett.116.063004 Phys. Rev. Lett. 116, 063004 (2016)] | ||
+ | #B. Hemmerling, E. Chae, A. Ravi, L. Anderegg, G. K. Drayna, N. R. Hutzler, A. L. Collopy, J. Ye, W. Ketterle and J. M. Doyle, '''Laser slowing of CaF molecules to near the capture velocity of a molecular MOT''', [http://iopscience.iop.org/article/10.1088/0953-4075/49/17/174001/meta J. Phys. B 49, 17 (2016)] | ||
+ | #E. Chae, L. Anderegg, B. L. Augenbraun, A. Ravi, B. Hemmerling, N. R. Hutzler, A. L. Collopy, J. Ye, W. Ketterle, and J. M. Doyle, '''One dimensional magneto-optical compression of a cold CaF molecular beam''', [https://arxiv.org/pdf/1701.03254v1.pdf arXiv:1701.03254v1] |
Latest revision as of 11:38, 13 January 2017
In the laser cooling lab, we seek to study ultracold molecules by first loading molecules into a magneto-optical trap (MOT) and then transferring these molecules to a magnetic trap for further cooling. Interesting later experiments could involve studying atom-molecule or molecule-molecule collisions, as well as using the ultracold sample of diatomic molecules for quantum simulation or precision measurement experiments.
Research Overview
The goal of this experiment is to load a magneto-optical trap (MOT) with the diatomic radical calcium monofluoride (CaF) using a two-stage buffer-gas beam source (for details on buffer-gas cells see [1-3]). We first ablate a solid precursor of atomic Ca with a pulsed Nd:YAG laser. We simultaneously flow sulfur hexafluoride (SF6) into the buffer-gas cell, leading to a chemical reaction which produces CaF. The hot molecular gas then thermalizes with ~1 K Helium buffer-gas and is extracted into a beam. The molecular beam has an average forward velocity of 50-60 m/s out of our two-stage cell. While such velocities are low enough to load conventional atomic MOTs (see our previous work on lanthanide atoms), the estimated capture velocity for a MOT of CaF is less than 10 m/s. A slowing stage is thus required to bring a sufficient number of molecules to below the capture velocity. We use a white-light slowing technique for this beam deceleration, as was demonstrated in our recent paper [14]. An additional challenge to trapping molecules is the existence of magnetic dark states in molecules, which arise due to the fact that we trap the molecules on a transition with "inverted" angular momentum structure. We address this problem by switching the polarization and the magnetic field of the MOT very rapidly (~1 MHz) to depopulate those dark states (see below for details).
Latest News
(August 2016) CaF slowing results published in J. Phys. B
Recently, we have accomplished laser slowing of CaF molecules from a two-stage buffer gas beam source down to longitudinal velocities of approximately 5 m/s. Our scheme uses three lasers (one main transition and two for repump transitions) which are spectrally broadened to address the velocity spread of the source as well as the hyperfine structure of the molecules. Our work on laser slowing of CaF has been published in J. Phys. B: At. Mol. Opt. Phys. for a special issue on "Atomic and molecular processes in the ultracold regime, the chemical regime, and astrophysics." You can find the paper here.
(November 2015) Eunmi defends her thesis
Congratulations to Eunmi Chae for successfully defending her thesis! Eunmi will continue her work on laser slowing and cooling of CaF for a few months before starting a post doc in the Takahashi group at Kyoto.
(Summer 2014) AC-MOT of Lithium
We realized an AC-MOT for Lithium-6 on the D1 line. See the picture on the right for an image of the MOT in action. This transition has magnetic dark states which reduce the efficiency of a regular DC-MOT configuration. A switching of the polarization and the magnetic field actively remixes the Zeeman substates and keeps the atom in the cooling cycle. Three different time traces comparing the beam signal without trapping fields, a DC-MOT and an AC-MOT are shown in the left figure. Both the magnetic field coils and the polarization of the molasses laser beams are switched at a frequency of 6 MHz. The improvement in the lifetime using the AC configuration is quite evident. For further details on AC-MOTs see e.g. Phys. Rev. Lett. 110, 143001 (2013) and Phys. Rev. Lett. 101, 173201 (2008).
(June 2014) Atomic MOTs paper accepted in New Journal of Physics
Our paper Buffer-gas loaded magneto-optical traps for Yb, Tm, Er and Ho has been accepted in New Journal of Physics.
People
Grad Students
- Loic Anderegg
- Ben Augenbraun
Former Students and Postdocs
- Aakash Ravi
- Eunmi Chae
- Boerge Hemmerling
- Nick Hutzler
- Garrett Drayna
Collaborators
- Jun Ye (PI), Matthew Hummon, Mark Yeo, Alejandra Collopy, Yong Xia
Ye Group Ultracold Molecules Website
- Wolfgang Ketterle (PI)
Detailed Overview of Experiment
We are investigating the laser slowing and magneto-optical trapping of diatomic molecules. Laser cooling of molecules requires buffer-gas cooling to produce samples of ground-state molecules which have necessary rotational phase space densities for current molecular laser cooling schemes. We use slow beam technology developed in our group [1-3] to reduce the amount of kinetic energy and number of cycled photons needed to bring molecules to rest in a 3D trap.
Currently, our group is working with the radical CaF. We have produced a slow, cryogenic beam of CaF with rotational cooling and we see slowing of molecules down to longitudinal velocities of ~ 5 m/s, following a scheme similar to the one used in [3-6].
Other groups working on laser cooling and slowing of diatomic molecules include:
- CU Boulder: Yttrium oxide (YO). Jun Ye Group Ultracold Molecules Website
- Yale University: Strontium fluoride (SrF). Dave DeMille Group Website
- Calcium Fluoride: Calcium fluoride (CaF). Ed Hinds Group Website
Buffer-Gas Loaded MOTs for Ytterbium, Thulium, Erbium and Holmium
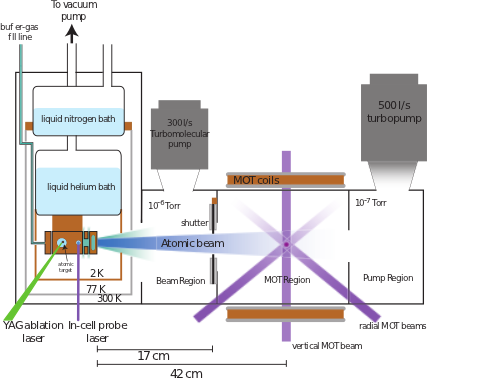
As a proof of principle for loading MOTs from a buffer-gas beam source, we first investigated laser cooling and trapping of atoms. We made the first buffer-gas loaded MOT using Yb atoms. A schematic of the apparatus can be seen on the right. This MOT, which used no slowing of any kind (Zeeman slower, slowing laser, etc.), demonstrated the feature of our buffer gas beam technology, which we plan to use for a molecular MOT. Furthermore, this MOT demonstrated that, in principle, buffer-gas beam technology is compatible with 3D magneto-optical trapping. Owing to the universal nature of this source, we implemented MOTs for the lanthanides ytterbium, thulium, erbium and holmium-- each requiring only a change of the ablation pre-cursor target and the MOT laser frequency. These highly magnetic species are of interest for quantum simulation and quantum computation. They are also refractory elements and have low loading rates due to the difficulties with oven sources. Our buffer-gas loaded MOTs circumvent these high temperature oven sources and allow loading rates over 10^8 /sec/shot, or over 10^9/sec cumulative. This technology could be used to co-load multiple species with little technological overhead (e.g., no multiple Zeeman slowers). We also demonstrate this flexibility in the case of ytterbium by loading many different isotopes into the MOT by adjusting the detuning of the MOT laser frequency only. Below is a video which shows a picture of the Yb MOT fluorescence with the detuning being changed between the frames.
Our work on these lanthanide atoms is summarized in the following article:
Buffer-gas loaded magneto-optical traps for Yb, Tm, Er and Ho. B. Hemmerling, G. K. Drayna, E. Chae, A. Ravi, and J. M. Doyle, New Journal of Physics 16, 063070 (2014).
Video of Ytterbium isotope MOTs
The solid precursor used for ablation contains the natural abundance of Yb isotopes, i.e. 3% 170Yb, 14% 171Yb, 22% 172Yb, 16% 173Yb, 32% 174Yb and 13% 176Yb. The ablation process, as opposed to photoionization, lacking an isotope-selective character brings each isotope into the gas phase where it is subsequently cooled by the He buffer gas. Due to the absence of a Zeeman slower, each element can be loaded into the MOT by only correcting for the isotope shift in the frequency detuning of the molasses laser. The top graph in the video shows the in-cell absorption spectrum and the change in laser frequency (The frequencies given in the video are measured with our wavemeter and thus are NOT precision measurements of the frequencies for the Yb transitions. For precise values, the reader is e.g. referred to PRA 82, 043408 (2010).) Note, that one of the isotopes, 173Yb, does not form a magneto-optical trap. This is due to the presence of magnetic dark states.
References
- N. R. Hutzler, H.-I Lu, and J. M. Doyle, The Buffer Gas Beam: An Intense, Cold, and Slow Source for Atoms and Molecules, Chem. Rev., 112, 4803 (2012)
- H.-I Lu, J. Rasmussen, M. J. Wright, D. Patterson, and J. M. Doyle, A cold and slow molecular beam, Phys. Chem. Chem. Phys. 13, 18986 (2011)
- D. Patterson and J. M. Doyle, Bright, Guided, molecular beam with hydrodynamic enhancement, J. Chem. Phys. 126, 154307 (2007)
- E. S. Shuman, J. F. Barry, D. R. Glenn, and D. DeMille, Radiative Force from Optical Cycling on a Diatomic Molecule, Phys. Rev. Lett. 103, 223001 (2009)
- E. S. Shuman, J. F. Barry, and D. DeMille, Laser cooling of a diatomic molecule, Nature 467, 820 (2010)
- M. T. Hummon, M. Yeo, B. K. Stuhl, A. L. Collopy, Y. Xia, and J. Ye, 2D Magneto-Optical Trapping of Diatomic Molecules, Phys. Rev. Lett. 110, 143001 (2013)
- M. Harvey, A. J. Murray, Cold Atom Trap with Zero Residual Magnetic Field: The ac Magneto-Optical Trap , Phys. Rev. Lett. 101, 173201 (2008)
- V. Zhelyazkova, A. Cournol, T. E. Wall, A. Matsushima, J. J. Hudson, E. A. Hinds, M. R. Tarbutt, and B. E. Sauer, Laser cooling and slowing of CaF molecules , Phys. Rev. A. 89, 053416 (2014)
- B. Hemmerling, G. K. Drayna, E. Chae, A. Ravi, J. M. Doyle, Buffer gas loaded magneto-optical traps for Yb, Tm, Er and Ho, New J. Phys. 16, 063070 (2014)
- J. F. Barry, D. J. McCarron, E. B. Norrgard, M. H. Steinecker, and D. DeMille, Magneto-optical trapping of a diatomic molecule, Nature 512, 286 (2014)
- D. J. McCarron, E. B. Norrgard, M. H. Steinecker, D. DeMille, Improved magneto-optical trapping of a diatomic molecule, New J. Phys. 17, 035014 (2015)
- M. Yeo, M. T. Hummon, A. L. Collopy, B. Yan, B. Hemmerling, E. Chae, J. M. Doyle, J. Ye, Rotational state microwave mixing for laser cooling of complex diatomic molecules, Phys. Rev. Lett. 114, 223003 (2015)
- E. B. Norrgard, D. J. McCarron, M. H. Steinecker, M. R. Tarbutt, and D. DeMille, Submillikelvin Dipolar Molecules in a Radio-Frequency Magneto-Optical Trap, Phys. Rev. Lett. 116, 063004 (2016)
- B. Hemmerling, E. Chae, A. Ravi, L. Anderegg, G. K. Drayna, N. R. Hutzler, A. L. Collopy, J. Ye, W. Ketterle and J. M. Doyle, Laser slowing of CaF molecules to near the capture velocity of a molecular MOT, J. Phys. B 49, 17 (2016)
- E. Chae, L. Anderegg, B. L. Augenbraun, A. Ravi, B. Hemmerling, N. R. Hutzler, A. L. Collopy, J. Ye, W. Ketterle, and J. M. Doyle, One dimensional magneto-optical compression of a cold CaF molecular beam, arXiv:1701.03254v1